- By Natalie Strehlke
- Automation Basics
Summary
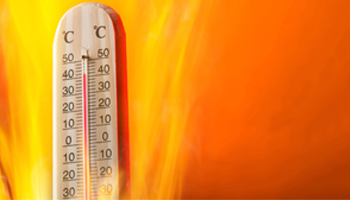
Natalie Strehlke
Thermocouple selection
A thermocouple is a closed-circuit thermoelectric temperature-sensing device consisting of two wires of dissimilar metals joined at both ends. A current is created when the temperature at one end or junction differs from the temperature at the other end. This phenomenon is known as the Seebeck effect, which is the basis for thermocouple temperature measurements.
One end is referred to as the hot junction, and the other end is referred to as the cold junction. The hot-junction measuring element is placed inside a sensor sheath and exposed to the process. The cold junction, or the reference junction, is the termination point outside the process where the temperature is known and where the voltage is being measured. This termination point is usually a control system input card, or a temperature transmitter.
There are many types of thermocouples made up of various metal combinations. These combinations have different output characteristics that define the applicable temperature range and the corresponding voltage output. The higher the magnitude of the voltage output, the higher the measurement resolution, which increases repeatability and accuracy (figure 1).
Figure 1. T/C EMF versus temperature curves. Repeatability and accuracy can be improved by selecting a thermocouple with a higher voltage output per degree of measurement.
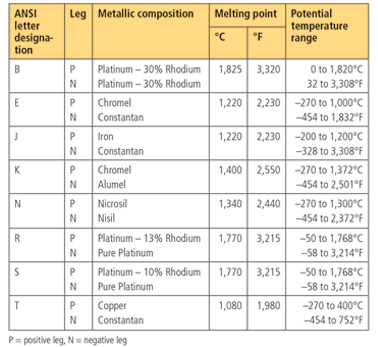
Figure 2 shows the temperature ranges of various thermocouple types along with other important characteristics, such as melting point and metallic composition. Type K is one of the most common general-purpose thermocouples, with a sensitivity of approximately 41 μV/°C. It is inexpensive; its potential range is –270°C to +1,372°C; and it is relatively linear.
Type J thermocouples have a more restricted potential range than type K at –200°C to +1,200°C, but a higher sensitivity of about 50 μV/°C. These thermocouples are very linear in the range of 149°C to 427°C.
Type E thermocouples have a potential range of –270°C to 1,000°C, and have the highest output versus temperature change of any standard thermocouple type at 68 μV/°C.
The type N thermocouple is a relatively new design and achieves considerably higher thermoelectric stability than the base-metal types E, J, K, and T. Type N thermocouples have a sensitivity of 39 μV/°C, a potential range from –270°C to 1,300°C, and have been used reliably for extended periods at temperatures up to at least 1,200°C. Type R and type S thermocouples have a potential range of –50°C to 1,768°C, but long-term high-temperature exposure can lead to mechanical failure and a negative calibration drift. Type B thermocouples have a potential range from about 0°C to 1,820°C, and suffer less from the high-temperature exposure issues affecting type R and S thermocouples.
Given these parameters, type K thermocouples are often the best choice for measuring temperatures up to about 1,000°C. Type J thermocouples are typically not used due to their nonlinearity at higher temperature ranges. Type E thermocouples are often applied in up to 1,000°C environments with high accuracy and repeatability requirements due to their very high output versus temperature change of 68 μV/°C.
All of these base metal types suffer to some degree from poor thermoelectric stability at elevated temperatures, leading to more widespread use of type N thermocouples in high-temperature
applications. As compared to type R, S, and B thermocouples, type N designs have a higher output versus degree of temperature change, and are thus generally more accurate and yield more repeatable measurements. After picking the right thermocouple type, the next step is thermowell selection.
Thermowell selection
Temperature sensors are rarely inserted directly into an industrial process, but are instead installed into a thermowell to isolate them from the potentially damaging process conditions of flow-induced stress, high pressure, and corrosive chemical effects.
Thermowells are closed-end metal tubes installed into the process vessel or piping. They become a pressure-tight, integral part of the process vessel or pipe. To select a proper thermowell, the process conditions must be identified, because they influence decisions regarding material of construction, well design, insertion length, and required lagging.
For high-temperature measurement applications, the thermowell's material of construction is very important. Figure 3 shows the maximum operating temperature for various materials and can be used as a guide for selecting the right thermowell for the application.
Figure 3. Maximum operating temperatures of thermowell materials. Thermowells come into direct contact with the process media, so it is critical to select the right construction material for high-temperature applications.
Thermowell selection can be very complex, particularly for high-temperature measurement applications, and will not be discussed in detail in this article. Please see the third resource for more information about thermowell selection or consult with your temperature measurement system supplier.
Transmitter selection
A thermocouple can be wired directly back to an input module on a control system, but this approach is not typical. The millivolt thermocouple signals are very susceptible to electrical noise, and thermocouple wiring is quite expensive.
For these and other reasons, it is considered best practice to connect the thermocouple wiring to a local transmitter, which amplifies the sensor's low-level mV signal to a more robust 4“20 mA current signal or to its digital equivalent in the case of a smart transmitter.
Transmitters used in high-temperature measurement applications are no different than those used to measure lower temperatures, because the transmitter is not subject to the process media as is the thermowell and, to a lesser extent, the thermocouple. But temperature measurement applications in general often require measurement at multiple points in a short-length pipe or a single vessel. For these applications, high-density transmitters are often used to accept multiple thermocouple outputs and to send one digital output signal to the control system.
For example, the transmitter shown in figure 4 can accept up to eight thermocouple inputs and transmit the measurement information from each via a single FOUNDATION Fieldbus cable. A different model of the same transmitter can accept up to four thermocouple inputs and transmit their measurement information via a WirelessHART network. Up to 16 of these transmitters can be interconnected, allowing 128 measurements to be sent to the control system via FOUNDATION Fieldbus or 64 measurements via WirelessHART.
Figure 4. This Rosemount 848T temperature transmitter accepts up to eight thermocouple inputs and transmits measurement information from each via a single FOUNDATION Fieldbus cable.
Other alternatives
Thermocouples mounted in thermowells are the most common way to measure high temperatures in process plants, but various noncontact temperature measurement methods are also available. These methods do not require process penetrations or thermowells, and can be much simpler to design, install, and maintain for these reasons. Resource five describes these noncontact temperature measurement methods in detail.
Although these methods have advantages, their use has been limited to applications that do not require a quick response or high accuracy. But a new method of noncontact temperature measurement provides traditional noncontact temperature measurement technologies with the quick response and high accuracy of insertion methods (figure 5). Although a high-temperature version of this technology is not available yet, it is expected in future product releases.
Selecting the right high-temperature measurement solution can be challenging, but many online resources exist to aid efforts, some of which are listed in the resources section. Suppliers of these systems can also assist in many cases.
Figure 5. Noncontact instruments like this Rosemount X-Well hold promise for measuring high-temperature process media, as no thermowell is required.
Reader Feedback
We want to hear from you! Please send us your comments and questions about this topic to InTechmagazine@isa.org.